10 Enzyme Kinetics
1. Objectives
- Enzymes as biological catalyst
- Enzyme Catalysis
- Understanding enzyme Kinetics
- Equations for enzyme kinetics
- Multisubstrate system
- Enzyme Inhibition
2. Enzymes
Enzymes (proteins) catalyze a chemical reaction that takes place within a cell (but not always). All proteins, including enzymes are synthesized by ribosomes.
Enzymes are of primary importancein carrying out metabolic pathways, which otherwise would require high amount of energy (heat) in processing chains of chemical reaction, but enzymes (highly specific for substrate and reaction type)help to pull off these reactions at higher rate and at mild temperature and pressure.
An enzyme by joining with its specific substrate lowers the energy required for activation of that particular reaction (Activation energy). The reaction occurs, and the enzyme is released again unchanged to be used again.
3. Enzyme catalysis
CATALYST: it can be defined as any substance which performs its function by enhancing the reaction rate without itself being consumed as after the reaction completes, catalyst is now free for another reaction. A reaction which is driven by a catalyst is termed as catalyzed reaction, and the process is known as catalysis. Catalyst has certaincharacteristics described below:
- It provides a different mechanism for the reaction and lowers the Gibbs energy of activation.
- Initially an intermediate is formed with the reactant(s) by a catalyst which is released later on during the product formation step.
- The catalyst cannot alter the thermodynamic equilibrium constant, which means that it is not able affect the enthalpies or Gibbs energies of both the reactants and products.
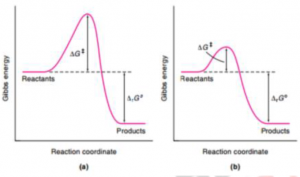
Fig.1 Gibb’s energy in (a) Uncatlyzed reaction. (b) Catalyzed reaction. ΔrGo remains same in both cases.
Enzymes escalate chemical reaction without itself being chemically transformed and they also do not alter the equilibrium of the reaction. Alternative reaction pathways with lower energy barriers are introduced by enzymes for catalysis. Catalytic Action: Enzyme accelerates the rate of the reaction without shifting or changing its equilibrium, and in order to understand this “Transition state theory” (Eyring, 1935) was given which suggests, Reactants must overcome an energy barrier and pass through an activated complex before moving on to the reaction products.
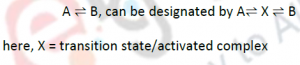
Enzyme introduces alternative pathways by following mechanism:
a.) Covalent catalysis: Catalytic functional group (nucleophile) attacks the substrate and forms covalent bond. Then electrons are withdrawn by an electrophilic catalyst resulting in rupture of the covalent bond, permitting further reaction and regeneration of enzyme based nucleophile. Eg. Decarboxylation of acetoacetate catalysed by primary amines.
b.) Acid base catalysis: A process involving partial proton transfer from an acid lowering the free energy of the transition state. A reaction is base catalysed, when the reaction rate is increased by partial proton abstraction by a base.Eg. Hydrolysis of peptide using chymotrypsin
c.) Proximity: The catalytic effectiveness is increased by bringing the substrate and catalytic groups closer (thereby increasing no. of collisions and reducing entropy. Eg. Imidazole-catalysed hydrolysis of esters, when the substrate and catalyst are brought in proximity to each other by hydrophobic interactions.
d.) Molecular distortion: The process in which the configuration of both enzyme and substrate, gets modified, upon binding of substrate is known as induced fit. Conformation of the substrate is changed in a way that it resembles the transition state; the conversion into the transition state is further facilitated by the stress created due to distortion of the substrate. The transition state is further stabilized by tight binding with enzyme and lowering activation energy requirement.
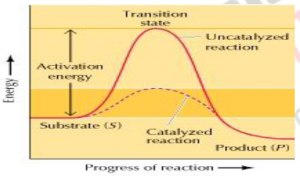
Fig.2Energy diagram representing catalyzed and uncatalyzed reaction. In presence of catalyst (enzyme) the activation energy is lowered (compare the peaks of catalyzed and uncatalyzed reaction).
4. Enzyme kinetics
Enzyme kinetics, deals with enzyme reactions which are time-dependent and explains the mechanisms of enzyme catalysis and its regulation.
Let’s understand enzyme kinetics as a function for the concentration of the substrate available for the enzyme.
- Start the experiment with a series of tubes which contains substrate, [S].
- At time (t) zero, addsome amount of the enzyme.
- Wait for few minutes
- Then, measure the newly formed product concentration. We can also use spectrophotometer, If product absorbs light.
- At a time when the amount of substrate is greater than the amount of enzyme, then, the rate is the initial velocity of Vi.
If we plot Vi as a function of [S], following observations will be made:
- At low [S], the initial velocity,Vi, rises linearly with increasing [S].
- When [S] increases, Vi settle down (rectangular hyperbolais formed).
- The asymptote shows Vmax as the maximum velocity of the reaction.
- The substrate concentration which produces a Vi equal to one-half of Vmax is called the Michaelis- Menten constant ( Km).
Km is (approximately) inversely related to the maximum reaction velocity, or strength of binding between the enzyme and its substrate. Lower the Km value, higher is the affinity for its substrate.
4.1 EQUATION OF ENZYME KINETICS
4.1.1 MICHAELIS MENTEN EQUATION
In 1913, Michaelis (1875–1949) and Menten (1879–1960, proceeded the work which was previously done by Frenchchemist V Henri (1872–1940), developed a mechanism to explain how the initial rate of enzyme- catalyzed reactions depends on the concentration.
Derivation of Michaelis-Menten equation: Few considerable assumptions can be made for the Michaelis- Menten equation derivation:
- Assuming that reverse reaction (P→ S) is negligible
- Assuming, there exists only a single central complex (ES). i.e. ES breaks down to E + P.
- Considering a situation when, [S] >> [E].Then the immediate interaction of S and E to form ES does not significantly affect free [S].
- Usually, ([S]-[ES])/[S] ≥ 99.9%
Considering the above assumptions, the reaction scheme is as follows:
Following are two parts for this reaction:
- Formation of ES complex (a second order process)
- The breakdown of ES complex to product P and free enzyme E (a first order process).
And the final Michaelis-Menten equation is as given below :
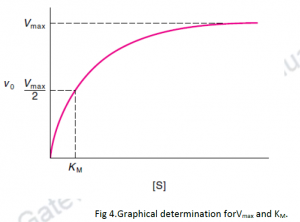
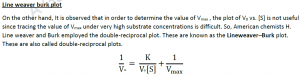
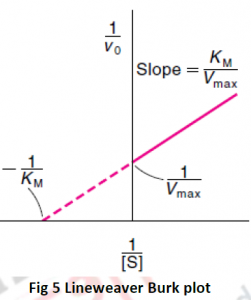
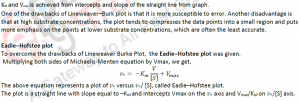
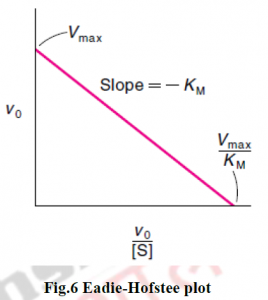
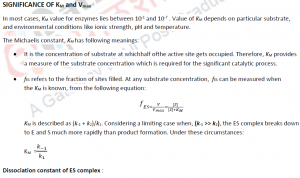
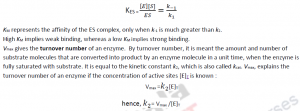
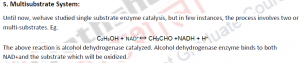
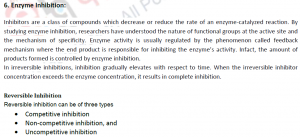
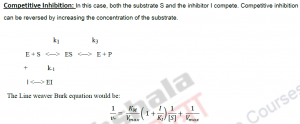
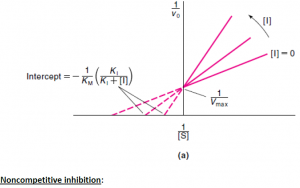
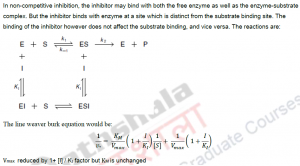
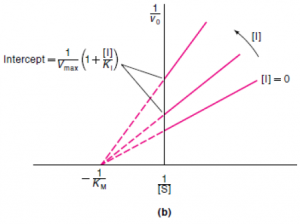
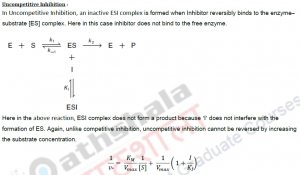
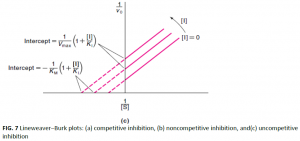